[Literature Review] Key Molecular Mechanisms of Prime Editing Revealed by Cryo-Electron Microscopy
A research team from the University of Tokyo, Japan, has published a study in the journal Nature titled "Structural basis for pegRNA-guided reverse transcription by a prime editor" with an impact factor of 50.5. This research utilizes cryogenic electron microscopy (cryo-EM) to reveal the low-temperature electron microscopic structures of the SpCas9-M-MLV RT ∆ RNaseH - pegRNA - target DNA complex in various states. The team designed pegRNA variants and Prime editor variants, truncated and fused the M-MLV RT, and successfully developed a smaller variant of the Prime editor, named PECO-Mini. This achievement paves the way for the development of a multifunctional Prime editing toolbox.
Original Article Link: https://doi.org/10.1038/s41586-024-07497-8
Prime editing is an advanced gene editing technology based on the CRISPR/Cas system, enabling precise fragment insertion, deletion, and arbitrary base substitution at target sites in the genome. It boasts higher accuracy and a lower error rate and has been widely applied to introduce precise mutations in human cells and various organisms. However, due to the lack of structural information, the mechanism underlying how the Prime editor recognizes and initiates the reverse transcription of the PBS-NTS heteroduplex priming and termination RTT (reverse transcription template) sequences remains poorly understood. To address this, researchers have determined the cryo-electron microscopy (cryo-EM) structures of the Prime editor under multiple states, providing a structural framework for understanding this innovative genome engineering system.
I.Defining the Structure of Prime Editor
Researchers purified the endogenous Prime Editor 2 (PE2) and conducted in vitro Prime editing experiments using pegRNA and 5’-cy5-labeled pre-nicked dsDNA substrates. PE2 generated DNA products through reverse transcription of the RTT sequence. In these experiments, pegRNA that stopped reverse transcription at the 5’ end of the RTT sequence was mixed with PE2, target DNA, and ddATP for incubation and assembly, allowing for the observation of the structural morphology of the Prime editor as it stalled during reverse transcription termination. Initial attempts may have been hindered by sample heterogeneity, resulting in the inability to obtain high-resolution cryo-electron microscopy density maps.
To overcome this issue, these researchers used a modified pegRNA with reduced byproduct production and replaced PE2 with purified catalytically inactive SpCas9 and engineered M-MLV RT∆RNaseH (RT∆RH), which was tested to exhibit editing efficiencies comparable to PE2. They used ddATP to reconstruct the state of the SpCas9-M-MLV RT∆RNaseH - pegRNA -target DNA complex at the time of reverse transcription termination, obtaining a three-dimensional (3D) reconstruction of the mock-up with an overall resolution of 3.0 Å, and a local refinement of RT∆RH to obtain a 3.5-Å local map. Finally, they combined these maps to generate a full model of the SpCas9–RTΔRH–pegRNA–target DNA complex.
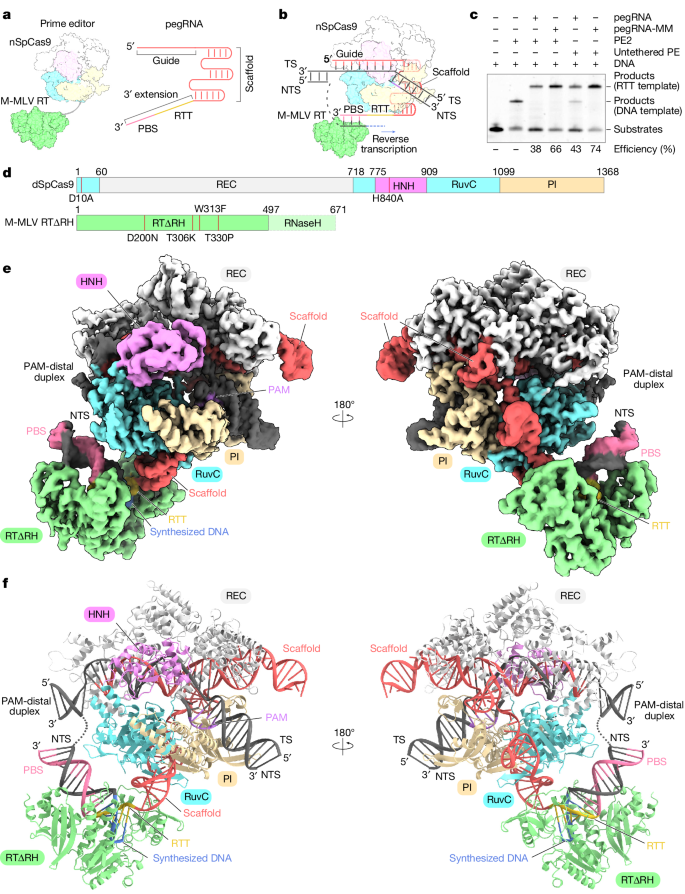
Figure 1: Cryo-EM Structure of the Prime Editor in the Termination State
II. Overall Structure of the Prime Editor
The cryo-EM structure reveals that SpCas9 assembles with a scaffold region of the pegRNA (G21–C96) to form a ribonucleoprotein and binds to the target DNA in a guide RNA-dependent and PAM-dependent manner. This suggests that the 3′ extension region of the pegRNA and M-MLV RT do not inhibit target DNA recognition by SpCas9. The 3' extension region forms an RNA-DNA heteroduplex with the NTS on the weakly positively charged surface that faces the SpCas9 RuvC structural domain. Additionally, nucleotides C103-G115 in the 3-nt PBS base pair form a PBS-NTS heteroduplex with nucleotides dC(-16*)-dG(-4*) in the NTS. The nucleotides C98-G102 in the 6-nt RTT form a base pair with the newly synthesized (reverse-transcribed) nucleotides. The dC1-3dG5 form a base pair on the NTS, and U97 at the 5' end of the RTT pairs with ddA6 in the NTS. In addition to the peripheral regions, RTΔRH, which binds to PBS-NTS and RTT-synthesized DNA heteroduplexes via a positively charged central groove, is clearly discernible in the density map. The M-MLV RT catalytic motif (YVDD) is situated in close proximity to the final U97-ddA6 base pair, suggesting that this structure represents the state in which the Prime editor has just completed reverse transcription up to the end of the RTT sequence.
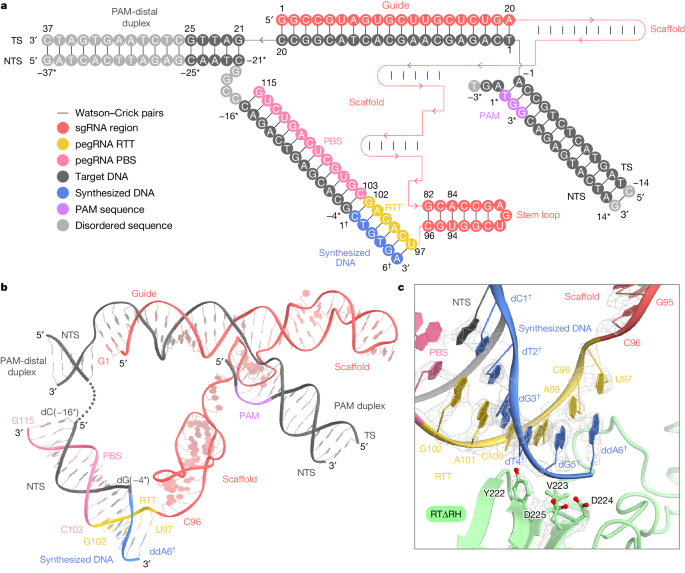
III. Extension of Reverse Transcriptase After the Termination Point of the RTT Sequence
The precise termination site and mechanism of reverse transcription in Prime editing using M-MLV RT, which can extend into the backbone region for pegRNA 3’ extensions, remain unclear. Through cryo-electron microscopy, researchers observed that while RTΔRH reverse transcriptase proceeds until the end of RTT, there is approximately a 10-Å separation between SpCas9 and RTΔRH. At the 3’ end of the scaffold region, C96 forms extensive interactions with key residues of RTΔRH, particularly stacking and hydrogen bonding between the ribose moiety of C96 and L99 and R116, respectively. Additionally, the G82-C96 base pair stacks with Y64, indicating that RTΔRH would continue reverse transcription beyond RTT into the backbone region if not halted by ddATP.
To biochemically characterize the reverse transcription termination site, researchers conducted in vitro Prime editing experiments using PE2 and non-contact PE. They observed that reverse transcription products with dNTPs were consistently 3 nucleotides longer than those with ddATP, suggesting that reverse transcription in PE2 and non-contact PE does not terminate at the end of RTT but progresses to U94 in the backbone region. These results indicate that M-MLV RT extends reverse transcription to U94 of pegRNA, three nucleotides upstream of RTT, and terminates reverse transcription by dissociating from pegRNA.
Prime editor induces backbone-derived short binding, resulting in non-desired editing at the target site. To eliminate these bindings, the researchers sought to design a pegRNA variant with a modified scaffold sequence exhibiting excessive reverse transcription. The design of pegRNA variants involves modifying U943-C96 to align with the target site and regulating A84-G82 to maintain the stem-loop structure. This approach effectively triggers pegRNA-dependent reverse transcription while eliminating scaffold-derived short binding. In vitro Prime editing experiments were conducted using wild-type pegRNA and three pegRNA variants with modified stem-loop sequences, and the results demonstrated that these modifications do not affect pegRNA-dependent reverse transcription activity. Furthermore, the researchers evaluated Prime editing efficiency in HEK293 cells using the PE2 and PE3 systems under five and four previously validated target conditions, respectively, and the findings indicated that the modified pegRNAs successfully induced the desired edits at a frequency comparable to that of wild-type pegRNAs.
Figure 3: Structural and Functional Analysis of Prime Editor in Terminated State.
IV. Process of Prime Editor Initiating Reverse Transcription
To investigate how the primer editor initiates reverse transcription, researchers designed a pegRNA that allows the incorporation of the first nucleotide, ddA. Using ddATP, the initial complex of SpCas9-RTΔRH-pegRNA-target DNA was reconstituted, resulting in a successful three-dimensional reconstruction with an overall resolution of 3.1 Å. Surprisingly, researchers observed a density corresponding to RTΔRH in this map, indicating that M-MLV RT occupies a fixed position relative to SpCas9 during reverse transcription initiation.
After local refinement of the RTΔRH-proximal region, a compositional map of the initial complex was obtained, and the characteristic Cα atoms of RTΔRH were identified, modeling its structure as a single rigid-body density. The map revealed that the PBS and NTS form an RNA-DNA heteroduplex, sandwiched between the RuvC domain of SpCas9 and RTΔRH. U102 at the 3' end of RTT forms a base pair with ddA1, while the remaining portion, except for G97 and A101, is disordered, indicating flexibility in RTT prior to reverse transcription.
Although RTΔRH is in a fixed position relative to SpCas9, it lacks direct interactions with the scaffold region of SpCas9 and pegRNA. Instead, RTΔRH forms extensive interactions with the PBS-NTS heteroduplex, and the active site is located near the U102-ddA1 base pair. These structural observations suggest that the positioning of the PBS-NTS heteroduplex may play a crucial role in determining the initiation site of reverse transcription.
To test this hypothesis, researchers reconstructed the Spcas9-pegRNA-target DNA complex without RTΔRH and attempted to determine the cryo-EM structure of its pre-initiation complex. Given that the length of the RTT sequence may limit the location of the PBS-NTS heteroduplex, researchers employed pegRNAs with extended RTT sequences for structural determination, thereby obtaining a three-dimensional reconstruction of the pre-initiation complex with a total resolution of 3.2 Å.
In the densitograms, it was observed that the PBS-NTS heteroduplexes corresponded to a rod density, indicating that the PBS-NTS heteroduplex is stably present on the surface. A comparison of the structures of the pre-initiation complex and the initiation complex indicates that the PBS-NTS heteroduplex is similarly located in both states. These structural observations indicate that the PBS-NTS heteroduplex is formed in proximity to the RuvC structural domain and that the M-MLV RT subsequently recognizes and binds this heteroduplex to initiate reverse transcription of the RTT sequence.
To capture the elongation state of the Prime editor, researchers prepared a pegRNA with a 28-nt RTT designed to terminate reverse transcription with ddATP at the 16th nucleotide and analyzed its structure by cryo-electron microscopy. By employing 3D sorting and local refinement, they were able to obtain a complex density map of the extended complex (16-nt) from the overall map at 3.1 Å resolution and the localized map near the RTΔRH at 6.1 Å resolution. In the latter, the density corresponding to the long RNA-DNA heteroduplex was observed on the surface of the RuvC structural domain, which binds the RTΔRH at the end.
A comparison of the structures of the initiation and extension complexes revealed that the alignment of RTΔRH relative to SpCas9 remained largely unaltered despite 15 nt of reverse transcription progression. This observation indicates that the M-MLV RT maintains a consistent position in relation to the initiation site throughout the reverse transcription of the RTT. Researchers put forth a daring hypothesis that M-MLV RT tethered within SpCas9 via a brief linker to anchor it in proximity to the initiation site would effectively induce pegRNA-dependent reverse transcription.
To test this hypothesis, researchers engineered two PE2 variants in which RTΔRH was inserted into the RuvC structural domain between G1054 and E1055 or between T1068 and G1069. These variants were purified and found to have reverse transcription efficiencies comparable to those of wild-type PE2. Furthermore, structural comparisons revealed that the formation of RTT-synthesized DNA heteroduplexes caused the PBS-NTS heteroduplex to be pushed in the opposite direction to RTΔRH, resulting in a rearrangement of PAM-distal target DNA duplexes. These structural and biochemical observations indicate that M-MLV RT consistently performs reverse transcription of RTT sequences at the initiation site and that RTT-synthesized DNA heteroduplexes are established along the longitudinal surface of SpCas9, resulting in rearrangement of PAM-distal target DNA duplexes.
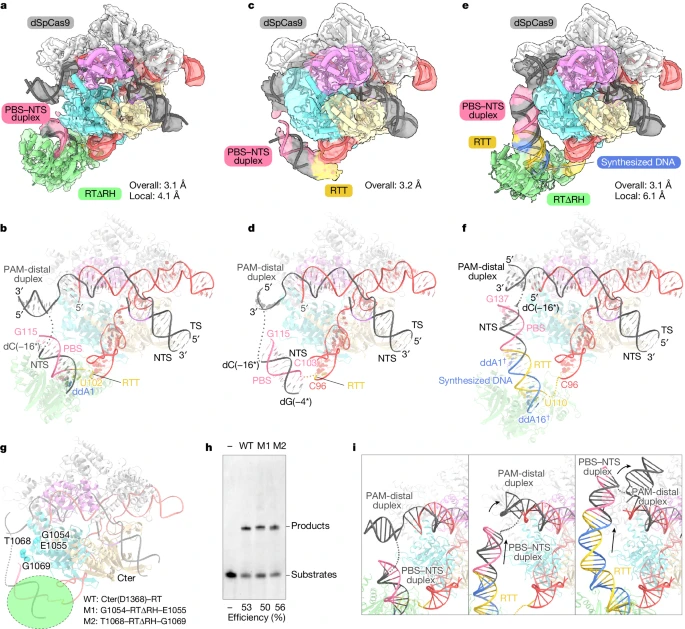
Figure 4: Cryo-EM Structures of the Prime Editor in Multiple States.
In conclusion, this study elucidates the structural configuration of Prime editor in various states when complexed with SpCas9, M-MLV RT, pegRNA, and target DNA, as observed through cryo-electron microscopy (cryo-EM) structural analysis. Furthermore, it employs rational design techniques to create pegRNA variants and Prime editor variants, provides structural insights into the step-by-step mechanism of Prime editing, and has established a foundation for the development of a versatile Prime editing toolbox.
EDITGENE, leveraging Prime Editing technology , has comprehensively upgraded its Bingo™ platform for site-specific mutagenesis. Drawing from extentive experiences in thousands of CRO projects, EDITGENE has optimize its technical approach, resulting in significantly higher success rates compared to conventional PE methods.
EDITGENE now offers precise and efficient gene point mutation cell line construction services to a broad spectrum of scientific research enterprises and communities.
Recent blogs:
1.[Star of the Month] Human Genome KO Library,Mouse Kinome CRISPR KO Library,Yeo Lab RNA-binding Protein CRISPR KO Library
2. [Literature Review] The CRISPRi Screen Helps Find Key Genes that Regulate Glioblastoma
Follow us on social media
Contact us
+ 833-226-3234 (USA Toll-free)
+1-224-345-1927 (USA)
info@editxor.com
Comment (4)