EDITGENE CO., LTD

17800 Castleton St. Ste 665. City of Industry. CA 91748

info@editxor.com

+1-833-226-3234 (USA Toll-free)
+1-224-345-1927 (USA)
+86-19120102676 (Intl)
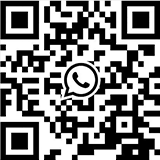
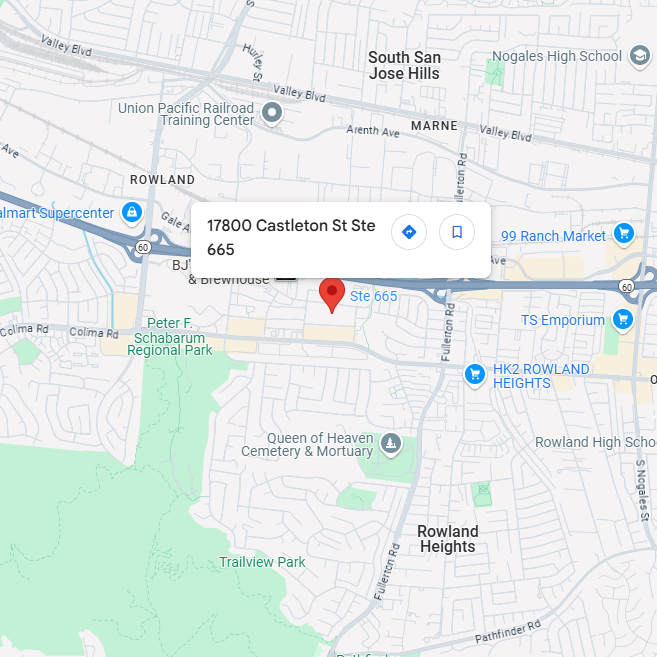
Technical Support
Support Center
Beijing Time: Monday to Friday, 8:00 AM - 6:00 PM
Toll-Free (USA): +833-226-3234
Direct Line (USA): +1-224-345-1927
Email: techsupport@editxor.com
After-Hours Support
Beijing Time: Monday to Sunday, 8:00AM - 6:00 PM
International Line: +86-19120102676
Email: info@editxor.com
Whatsapp
Connect with us instantly on WhatsApp for quick inquiries and real-time support.
Office WhatsApp
Facebook Messenger
Reach out to us on Facebook Messenger for personalized assistance and detailed information.
Linkedin
Engage with us on LinkedIn for professional inquiries, the latest blogs, discoveries, and updates on our innovative work.
FAQ
Why choose EDITGENE?
EDITGENE provides access to a comprehensive library of over 4,500 high-quality knockout (KO) cell lines, enabling researchers to save valuable time. Our custom gene knockout services are highly efficient, boasting a high positive rate while minimizing off-target effects. Clients also benefit from personalized, one-on-one support from a team of PhD experts from globally renowned institutions, ensuring top-tier service and results.
Why do researchers use KO cell lines?
Researchers use KO cell lines to investigate gene functions by observing the effects of gene deletion on cellular behavior. This helps in understanding the role of genes in various processes like cell growth, metabolism, and signal transduction. KO cell lines are vital for studying diseases like cancer, genetic disorders, and neurodegenerative diseases.
Are all types of genes suitable for KO cell lines?
Not all genes are suitable for knockout. Some gene knockouts may result in cell death or severe dysfunction, particularly for essential genes. In such cases, conditional knockouts or gene knockdowns (e.g., RNAi) may be used instead.
Why choose EDITGENE to establish stable overexpression cell lines?
EDITGENE brings 10 years of CRISPR-based cell editing experience and offers one-on-one support from a team of PhDs from globally recognized institutions.
How to Improve the Detection Sensitivity of Cas Enzymes?
1.Design an efficient crRNA sequence. Proper design and structure prediction using online resources can help select suitable crRNA to achieve good trans-cleavage activity of the Cas enzyme.
2.Choose an appropriate signal reporter substrate. Research indicates that using a 15 nt single-stranded DNA (ssDNA) as a reporter substrate maximizes the cleavage reaction rate of Cas12a, significantly enhancing the reaction rate compared to the commonly used 5-nt ssDNA.
3.Optimize reaction conditions and buffers. Adjusting the CRISPR reaction parameters, such as the ratio of Cas enzyme to crRNA, the concentration of the Cas enzyme, and the reaction temperature, can improve detection performance to some extent.
2.Choose an appropriate signal reporter substrate. Research indicates that using a 15 nt single-stranded DNA (ssDNA) as a reporter substrate maximizes the cleavage reaction rate of Cas12a, significantly enhancing the reaction rate compared to the commonly used 5-nt ssDNA.
3.Optimize reaction conditions and buffers. Adjusting the CRISPR reaction parameters, such as the ratio of Cas enzyme to crRNA, the concentration of the Cas enzyme, and the reaction temperature, can improve detection performance to some extent.
What issues should be considered when culturing cells for gene delivery?
Maintaining the activity of cell cultures is crucial. Cells should not be allowed to reach confluence for more than 24 hours. Frozen new cells can restore transfection activity. The optimal cell plating density varies for different cell types or applications; however, for adherent cells, a confluence of 70% to 90% or a density of 5×10^5 to 2×10^6 suspended cells/ml typically yields good transfection results. It is important to ensure that cells are not fully confluent or in a fixed phase during transfection.
What is lentivirus packaging?
Lentivirus is a gene delivery tool that introduces exogenous genes into cells. By using tool cells such as 293T, lentiviral vectors carrying target DNA fragments are packaged into lentiviral particles with cell-infectious activity. This packaging process includes constructing lentiviral vectors, preparing packaging plasmids, culturing tool cells, transfecting plasmids, collecting viral particles, purifying and concentrating viral particles, and titration.
What is the difference between a single-plasmid system and a dual-plasmid system for library vectors?
What is the difference between a single-plasmid system and a dual-plasmid system for library vectors?
A single-plasmid system can achieve gene editing with one transfection, making construction relatively simple, but the larger plasmid size can lead to lower infection efficiency. In a dual-plasmid system, two vectors are used, each carrying either the Cas9 or sgRNA expression cassette. A stable Cas9 cell line is first constructed, and then the sgRNA library is transfected into this cell line. This approach has several advantages:
1.Increased Editing Efficiency: The independent and stable expression of Cas9 protein and sgRNA on different vectors enhances editing efficiency.
2.Flexibility: Vectors can be designed and constructed flexibly based on experimental needs, such as loading two sgRNA expression cassettes into one vector.
3.Increased Viral Titer: By splitting into two plasmids, the load on each plasmid is reduced, facilitating viral packaging and increasing yield and titer.
4.Increased Stability: Independently constructing a stable Cas9 cell line ensures that the Cas9 expression levels and editing efficiency in each cell are approximately the same, enhancing experimental accuracy.
1.Increased Editing Efficiency: The independent and stable expression of Cas9 protein and sgRNA on different vectors enhances editing efficiency.
2.Flexibility: Vectors can be designed and constructed flexibly based on experimental needs, such as loading two sgRNA expression cassettes into one vector.
3.Increased Viral Titer: By splitting into two plasmids, the load on each plasmid is reduced, facilitating viral packaging and increasing yield and titer.
4.Increased Stability: Independently constructing a stable Cas9 cell line ensures that the Cas9 expression levels and editing efficiency in each cell are approximately the same, enhancing experimental accuracy.
How to Design crRNA?
1.The design process can follow these steps:
1.Identify the target gene sequence.
2.Specify the Cas protein being used. Different Cas proteins require corresponding PAM (Protospacer Adjacent Motif) sequences; for instance, Cas12a needs the "TTTV" PAM sequence for target recognition.
3.Select the crRNA targeting region. Choose a 20 nt nucleotide sequence on the target gene that is adjacent to the PAM site and pairs with the complementary strand of the crRNA.
4.Combine the selected 20 nt target sequence (variable part) with the scaffold sequence (fixed part) to design the crRNA sequence.
5.Use online tools such as CRISPR design tools (e.g., CRISPOR, Benchling, etc.) to assist in designing crRNA. These tools can predict the efficiency and specificity of the sgRNA, helping to avoid potential off-target effects.
6.After completing the design, the synthetic crRNA sequence can be ordered from a synthetic biology company.
1.Identify the target gene sequence.
2.Specify the Cas protein being used. Different Cas proteins require corresponding PAM (Protospacer Adjacent Motif) sequences; for instance, Cas12a needs the "TTTV" PAM sequence for target recognition.
3.Select the crRNA targeting region. Choose a 20 nt nucleotide sequence on the target gene that is adjacent to the PAM site and pairs with the complementary strand of the crRNA.
4.Combine the selected 20 nt target sequence (variable part) with the scaffold sequence (fixed part) to design the crRNA sequence.
5.Use online tools such as CRISPR design tools (e.g., CRISPOR, Benchling, etc.) to assist in designing crRNA. These tools can predict the efficiency and specificity of the sgRNA, helping to avoid potential off-target effects.
6.After completing the design, the synthetic crRNA sequence can be ordered from a synthetic biology company.
How does EDITGENE ensure the purity and stability of cells during monoclonal screening?
EDITGENE’s 3D single-cell printing technology employs non-contact operation, avoiding mechanical damage and background contamination, which helps maintain cell integrity and biological activity. This technology also minimizes human error in the traditional limited dilution method of monoclonal selection, ensuring the reliability of screening results.
What is the difference between KO cell lines and gene knockout animal models?
KO cell lines are used for in vitro experiments, suitable for high-throughput screening and cellular studies, while gene knockout animal models are used for in vivo experiments to study gene functions within an entire organism and its interaction with the environment.